4 Histology – Endocrine
Jeffrey Marchant, PhD
Endocrine Part I
Pituitary
gland
Learning Objectives
2) To describe the general concepts of endocrine gland development and the consequences of developmental anomalies (e.g. supernumerary or ectopic tissue).
3) To recall the different types of hormones produced by these endocrine glands and their main actions on target organs.
VOCABULARY
Acidophils | Mammotrophs | Thryotrophs |
Basophils | Pars distalis | |
Chromophobes | Pars Intermedia | |
Interstitial Cells | Pars Nervosa | |
Corticotrophs | Pars Tuberalis | |
Folliculostellate Cells | Rathke’s Pouch | |
Gonadotrophs | Somatotrophs | |
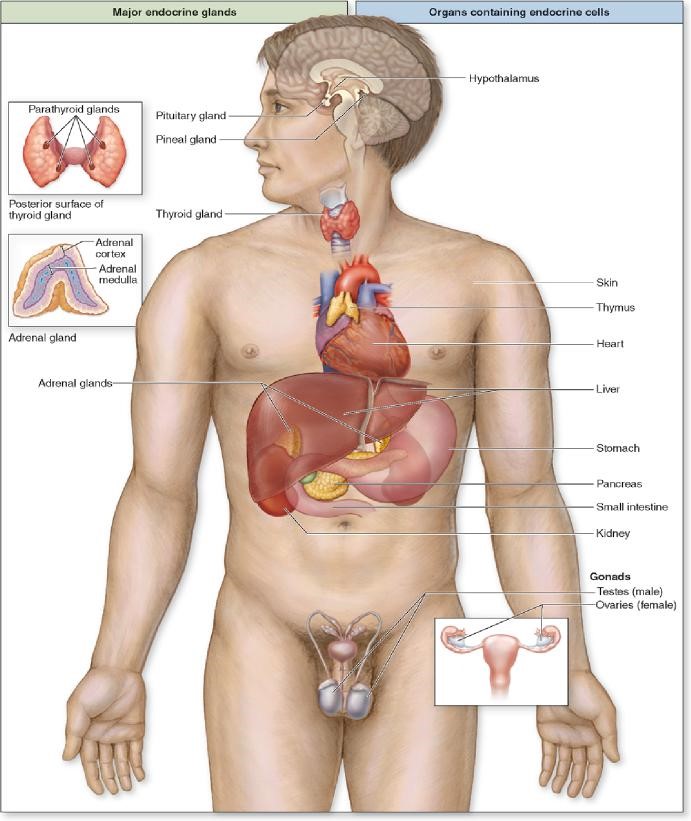
TEXTBOOK CHAPTERS
Wheater’s Functional Histology: Chapter 17. Endocrine System
Junqueira’s Basic Histology: Chapter 20. Endocrine Glands
OVERVIEW
The endocrine glands consist of diverse tissues and organs that coordinate the activity of other body tissues and organs by means of specific molecules called hormones. These endocrine signals are transported in the blood and other body fluids, often bound to specific binding proteins, to their targets (cells, tissues or organs) where they exercise a particular effect or response via specific receptors. Some endocrine glands are under CNS influence, whereas others respond to changes in their surroundings. All are highly vascular and characterized by fenestrated capillaries or larger fenestrated sinusoidal capillaries.
In addition to endocrine glands, there are many hormones released from endocrine tissues that exist within a variety of organs. For example, renin and erythropoietin are excreted from the kidney, atrial natriuretic factor is produced in the heart atria, and secretin is made by enteroendocrine cells in the gut. These are just a few of many such examples of endocrine function in the body.
General functions of hormones include the regulation of biological clocks, contraction of smooth and cardiac muscle, gland secretion, and metabolism. In addition, hormones are important regulators of growth and development and reproduction.
Several classes of hormones exist including steroid, gas (e.g. nitric oxide), peptide and eicosanoid (derived from fatty acids).
Pituitary Gland
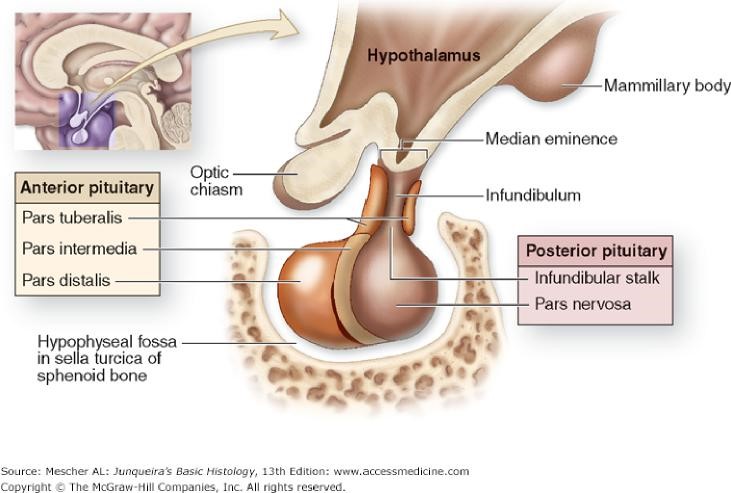
This gland regulates many other endocrine organs and thus is often called the “master” endocrine organ. It is located within a small bony cavity within the sphenoid bone Figure 2). The pituitary gland develops from both oral cavity tissues and neural tissue; consequently, there are several distinct parts in the adult organ (Figures 3 & 4). The neural tissue gives rise to the pars nervosa and infundibulum (these two neural-derived regions are often called the neurohypophysis or posterior pituitary, relating to its anatomical position); the oral tissue gives rise to the pars distalis, pars intermedia and pars tuberalis. Since the oral derivatives form an epithelial gland, another name for these regions, collectively, is the adenohypophysis (or the anterior pituitary). Each part serves a different function. The pituitary gland is controlled by the hypothalamus via the hypothalamohypophyseal tract, which directs the release of most pituitary hormones.
Pars Distalis
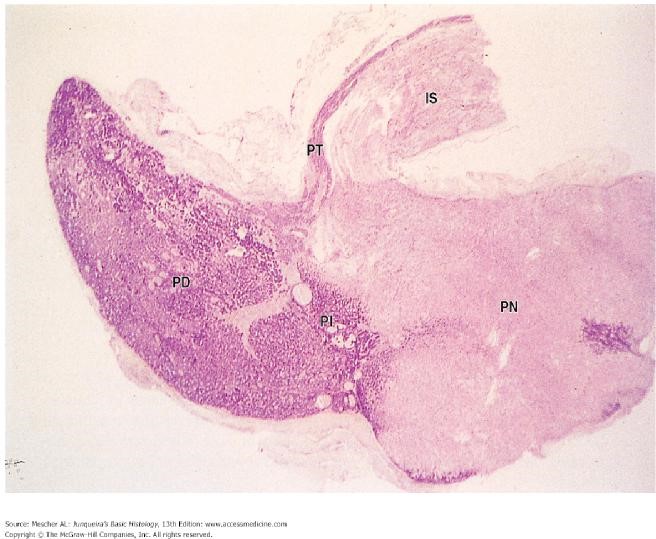
Histologically, three endocrine cell morphologies may be distinguished in this region (Figure 5): acidophils, which appear red due to their affinity for acidic dyes such as eosin; basophils, which appear blue due to their affinity for basic dyes such as hematoxylin; and chromophobes, which do not stain readily and remain pale in histologic preparations. Chromophobes may represent degranulated acidophils or basophils and it has also been suggested that they could be stem cells.
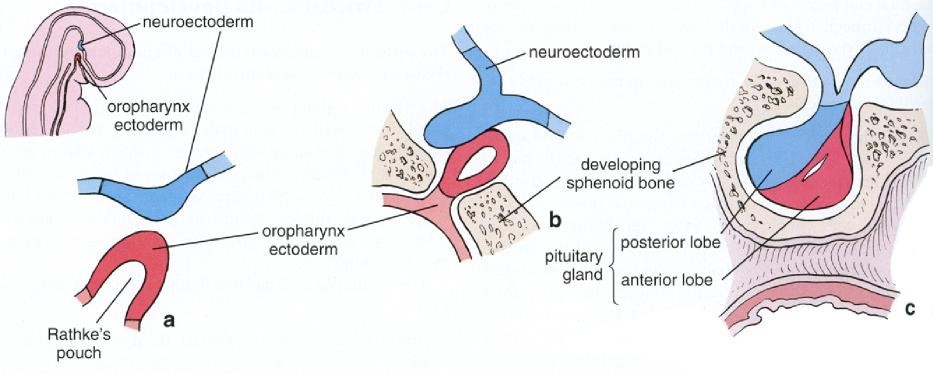
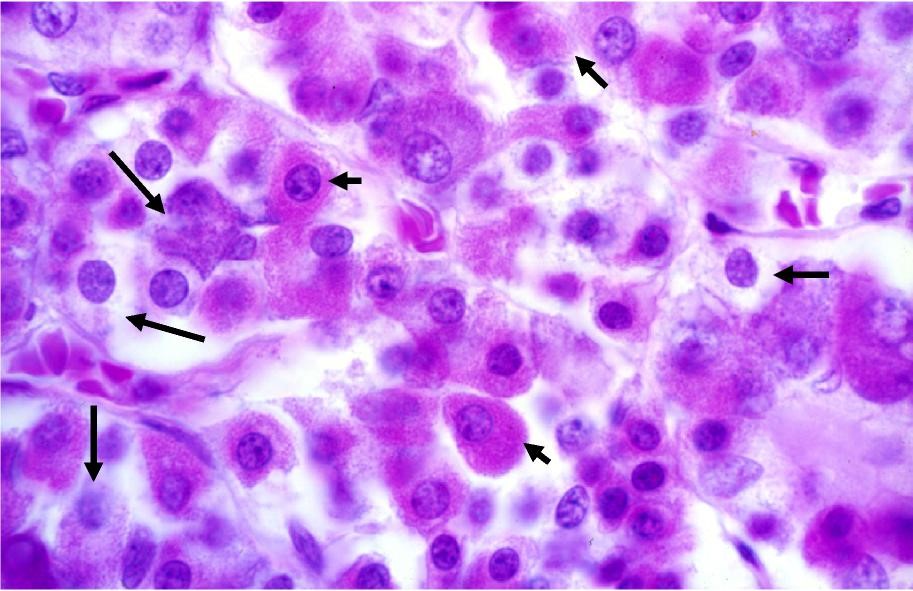
A total of five distinct cell types are present in the pars distalis:
1) Somatotrophs: these secrete growth hormone (GH) and account for 50% of the pars distalis cells. Release of GH is stimulated by growth hormone releasing hormone (GHRH) and inhibited by somatostatin from the hypothalamus. GH stimulates growth, cell proliferation and regeneration.
2) Mammotrophs: (also called lactotrophs) these secrete prolactin and account for 15-20% of the cells, however their numbers and size increase during pregnancy. These cells are stimulated by thyroid releasing hormone (TRH) and by vasoactive intestinal peptide. Prolactin stimulates milk production in the mammary gland.
3) Gonadotrophs: secrete follicle stimulating hormone (FSH) and luteinizing hormone (LH) and account for less than 10% of the total cell number. They are controlled by gonadotropin releasing hormone (GnRH) and luteinizing hormone releasing hormone (LHRH) from the hypothalamus. FSH stimulates germ cell maturation in both males and females. LH triggers ovulation in females and stimulates Leydig cells in males to produce testosterone.
4) Thyrotrophs: secrete thyroid-stimulating hormone (TSH), also called thyrotropin, and represent 5% of the cells. These are controlled by hypothalamic thyroid releasing hormone (TRH). TSH stimulates the thyroid gland to secrete thyroxine (T4) and triiodothyronine (T3).
5) Corticotrophs: secrete adrenocorticotropic hormone (ACTH) and are controlled by corticotropin releasing hormone (CRH). ACTH stimulates the cells of the adrenal cortex to release corticosteroids.
Somatotrophs and mammotrophs are acidophils, and gonadotrophs, thyrotrophs, and corticotrophs are basophils. These five types can be distinguished by TEM analysis.
In addition to the above cell types the pars distalis also contains specialized cells known as folliculostellate cells (Figure 6). These cells form an intercellular network between themselves and endocrine cells with numerous gap junctions. The function of these cells is not clear but they have been suggested to be involved in local control coordinating endocrine cell function or serving as a stem cell population.
A portion of the pars distalis extends superiorly toward the hypothalamus. This region forms a tube like cuff surrounding part of the posterior pituitary known as the infundibulum. This tube-like region is called the pars tuberalis.
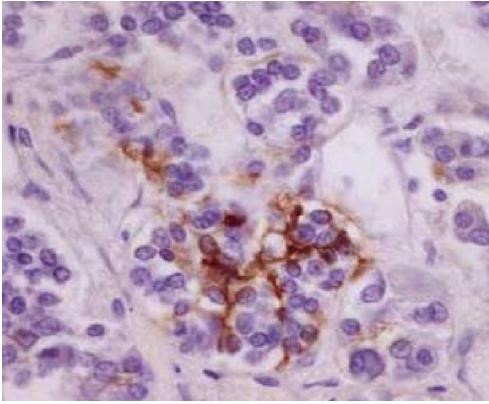
Table 1: Summary of cell types in the anterior pituitary and the hormones they produce. | |||
Type | % of Total Hormone Cells | Produced | Major Function |
Somatotrophs | 50% | Somatotropin (growth hormone, GH) | Stimulates growth in epiphyseal plates of long bones via insulin-like growth facors (IGFs) produced in liver. |
Lactotrophs | 15-20% | Prolactin (PRL) | Promotes milk secretion |
Gonadotrophs | 10% | Follicle-stimulating hormone (FSH) and luteinizing hormone (LH; interstitial cell-stimulating hormone [ICSH] in men) | FSH promotes ovarian follicle development and estrogen secretion in women and spermatogenesis in men; LH promotes ovarian follicle maturation and progesterone secretion in women and interstitial cell androgen secretion in men |
(mammotrophs) Thyrotrophs | 5% | Thyrotropin (TSH) | Stimulates thyroid hormone synthesis, storage, and liberation |
Corticotrophs | 15-20% | Adrenal corticotropin (ACTH) | Stimulates secretition of adrenal cortex hormones |
Lipotropin (LPH) | Helps regulate lipid metabolism |
Pars Nervosa
The posterior pituitary (PP) is derived from neural tissue and is also referred to as the neurohypophysis. It has three parts, the median eminence, infundibulum and pars nervosa. As a neural derivative, this tissue contains many unmyelinated axons as well as pituicytes (glial-like cells) and fenestrated capillaries Figure 7).
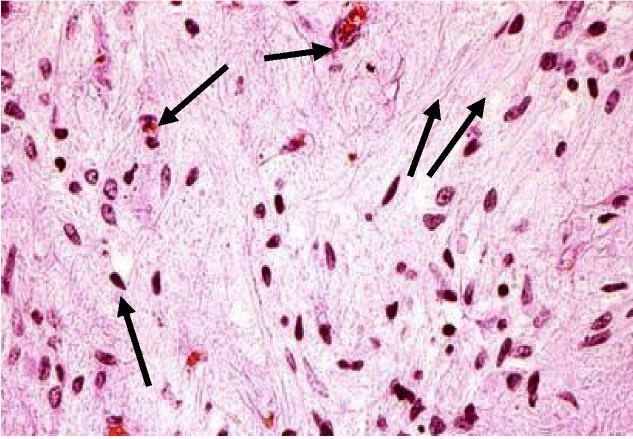
The pars nervosa contains axons extending from two hypothalamic nuclei: paraventricular and supraoptic, that release oxytocin and antidiuretic hormone (ADH, aka vasopressin). These hormones travel down axons to the pars nervosa and are stored in swellings near the axon terminus called Herring bodies (Figure 8). Thus, the pars nervosa releases these hormones in what is termed “neurosecretion” and is NOT involved in their synthesis The released hormones enter fenestrated blood vessels.
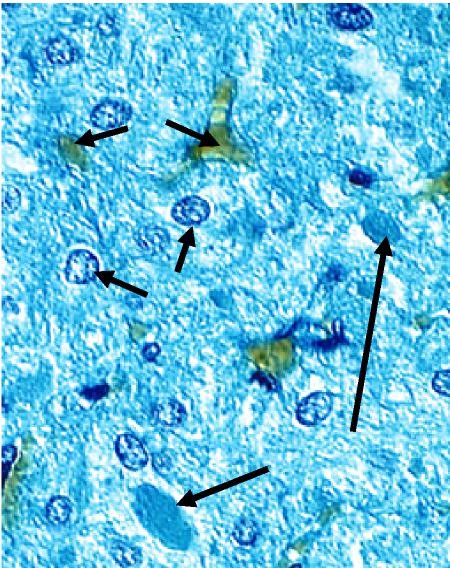
Pars Intermedia
The “intermediate lobe” lying between the pars distalis and pars nervosa, this lobe is derived from oral ectoderm and contains cords of cells that are basophilic. As such, this lobe resembles the pars distalis (Figure 9). Typically, this lobe is separated from the pars distalis by fluid-filled cysts that are remnants of Rathke’s pouch. Although not well developed in humans, these pars intermedia cells produce melanocyte stimulating hormone (MSH) from a large precursor protein, proopiomelanocortin. MSH stimulates production of the pigment melanin.
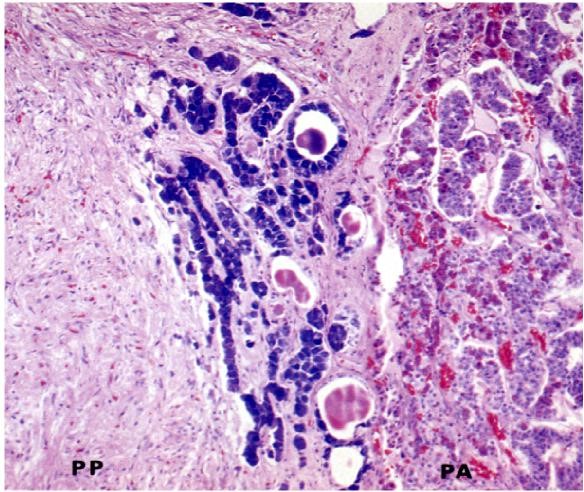
ENDOCRINE PANCREAS
The distribution of endocrine cells within each islet is not random: subsets of cells occupy different regions but are in close proximity to the fenestrated capillaries. Cells found in islets of Langerhans include α, 13, and δ cells. PP cells are a minor subset of endocrine cells that are found more often in islets near the head of the pancreas, which secrete pancreatic polypeptide. The pancreatic endocrine cells and their secretions are summarized in Table 2.
Table 2: Summary of Islet of Langerhans cells and the hormones they produce. | ||||
Cell Quantity | Hormone Produced | Hormone Structure and Size | Hormone Function | |
α | ∼20 | Glucagon | Polypeptide; 3500 Da | Acts on several tissues to make energy stored in glycogen and fat available through glycogenolysis and lipolysis; increases blood glucose content |
β | ∼70 | Insulin | Dimer of α and 13 chains with S-S bridges; 5700-6000 DA | Acts on several tissues to cause entry of glucose into cells and promotes decrease of blood glucose content |
δ o D | 5-10 | Somatostatin | Polypeptide; 1650 Da | Inhibits release of other islet cell hormones through local paracrine action; inhibits release of GH and TSH in anterior pituitary and HCl secretion by gastric parietal cells |
PP | Rare | Pancreatic Polypeptide | Polypeptide; 4200 Da | Stimulates activity of gastric chief cells; inhibits bile secretion,
pancreatic enzyme and bicarbonate secretion, and intestinal motility |
Diabetes mellitus
Diabetes mellitus is divided into insulin-dependent and non-insulin dependent (IDDM and NIDDM, respectively), also called type 1 and type 2 diabetes. In Type 1 diabetes (IDDM), an autoimmune response destroys 13 cells which is treated by insulin injections. Type 2 diabetes (NIDDM) results from target cell insulin insensitivity and 13 cells cannot produce enough insulin.
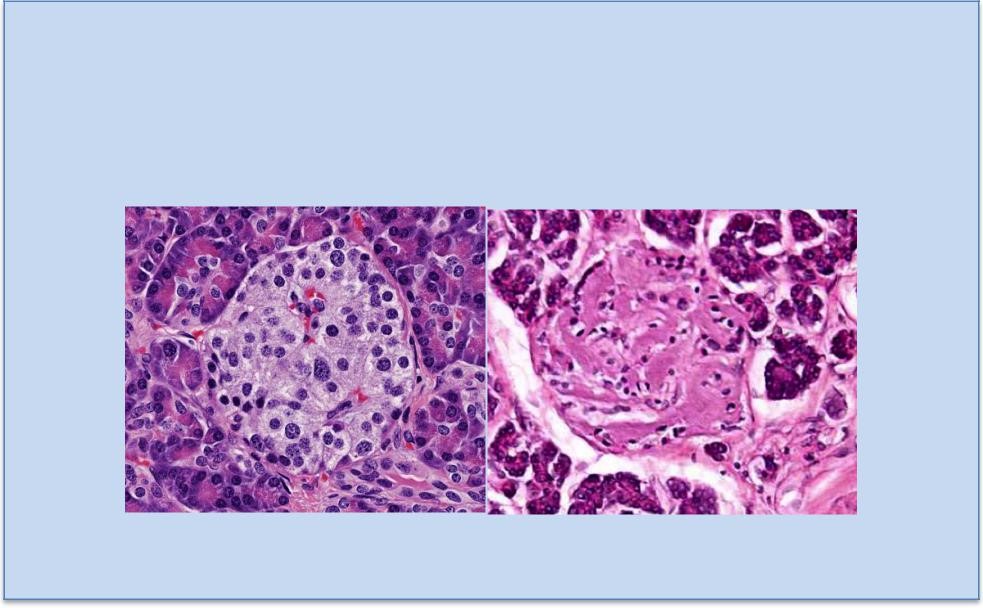
Endocrine Part II
Thyroid, parathyroid &
adrenal glands
LEARNING
OBJECTIVES
1)To identify the organization, function and histological appearance of these endocrine glands.
2) To describe the general concepts of endocrine gland development and the consequences of developmental anomalies (e.g. supernumerary or ectopic tissue).
3) To recall the different types of hormones produced by the endocrine glands and their main actions on target organs.
4) To describe the consequences of endocrine gland failure with respect to well-characterized diseases (e.g. Graves disease).
VOCABULARY
TEXTBOOK CHAPTERS
Wheater’s Functional Histology: Chapter 17. Endocrine System
Junqueira’s Basic Histology: Chapter 20. Endocrine Glands
OVERVIEW
In the previous chapter, several of the endocrine organs were discussed: the pituitary gland, the pineal gland, and the endocrine pancreas. These organs secrete hormones into the bloodstream that act on other tissues. This is in contrast to autocrine secretion, where a cell secretes products that act on itself; paracrine secretion, where a cell acts on neighboring cells without utilizing the bloodstream; and exocrine secretion, where cells secrete products through ducts to an external environment.
The thyroid, parathyroid, and adrenal glands also secrete their products directly into the bloodstream. This is made possible by the high degree of vascularization present in these glands, and the presence of fenestrated capillaries that carry secreted hormones quickly into the circulation and to their targets. These products are involved in a variety of processes, including metabolism, homeostasis, and fight-or-flight response.
Figure 2. The thyroid gland is located anteriorly and
surrounds the larynx
The thyroid gland is one of the largest endocrine glands in the body. It controls energy
utilization and is involved in control of growth and rate of function of many other systems of the body. It is controlled by thyroid secreting hormone (TSH), produced by the anterior pituitary, as seen previously. When stimulated by TSH, the thyroid produces triiodothyronine (T3) and thyroxine (T4), the latter of which participates in a negative feedback loop that suppresses the release of TSH from the pituitary. The body is very sensitive to changes in the activity of the thyroid, which will be discussed below.
Adjacent to the thyroid gland are the parathyroid glands. There are four of these glands, located posteriorly in the left and right lobes of the thyroid. The key role of the parathyroid is to control blood calcium levels and bone growth and resorption via production and release of parathyroid hormone, working with calcitonin released by the thyroid.
The adrenal glands are derived from neural crest and mesodermal tissue during embryonic development. They are involved in production of steroid hormones, including the catecholamines epinephrine and norepinephrine, and glucocorticoids such as cortisol.
THYROID GLAND
The thyroid gland is a butterfly-shaped organ in front of the trachea (Figure 2). It is derived from a downgrowth of oral epithelium from the base of tongue, forming the thyroglossal duct. In the oral cavity, all that remains of the duct after birth is a small structure called the foramen cecum. Occasionally, epithelial remnants of this down growth persist and can form cysts or ectopic glands. The gland is highly lobulated and each lobule consists of numerous follicles lined by a single layer of epithelial cells (Figures 3 & 6).
Figure 3. The thyroid follicle is filled with a thyroglobulin
colloid where thyroid hormone is stored in an inactive
form, which is resorbed and processed when hormone
is needed.
The lumen of each follicle is filled with a colloid made up of thyroglobulin, a precursor form of thyroid hormone. This hormone is synthesized via the following steps (and see Figure 4):
1) Thyrocytes produce thyroglobulin in the rER which is glycosylated in the Golgi. Thyroglobulin contains key tyrosine residues that are needed for functional thyroid hormone synthesis. Once synthesized, it is released into the follicular lumen from apical vesicles.
2) Iodide is taken up into the thyrocytes by Na/I symporters at the basolateral surface, which is then transported into the colloid at the apical surface.
3) Thyroid peroxidase on the luminal membrane iodinates tyrosyl residues of thyroglobulin
4) Formation of triiodothyronine (T3) and tetraiodothyronine (T4, also called thyroxine) occurs in the follicular lumen. These remain covalently bound to thyroglobulin in the colloid
5) Endocytosis of iodinated thyroglobulin occurs when thyroid hormones are needed. T3 and T4 are cleaved in lysosomes from thyroglobulin
6) T3 and T4 are secreted into adjacent capillaries at the basolateral surface of the thyrocytes.
Figure 4. Thyrocytes produce thyroglobulin, which they use to produce thyroid hormone from iodide that
they transport into the follicle lumen. When thyroid hormone is needed, the thyrocytes endocytose
thyroglobulin colloid, partially degrade it, and release the T3 and T4.
T3 is the more potent form of thyroid hormone and is active in the regulation of metabolic rate, heat production and growth and development.
Deep to the thyroid follicular epithelium reside parafollicular cells that synthesize and release the hormone calcitonin. These cells have a pale staining cytoplasm (hence, they are also known as “clear” or “C” cells, Figure 5). These cells produce calcitonin, a hormone that lowers blood calcium levels by lowering osteoclast activity and calcium absorption. Although pale-staining in histological sections, by TEM these cells are filled with calcitonin-containing granules (Figure 6). The clear cells’ location deep to the thyroid follicular cells is also evident when the cells are visualized by immunohistochemistry.
Figure 5. Clear cells (arrows) do not come into contact with the
follicular lumen. These cells secrete calcitonin, which acts to
decrease Ca2+ concentration in the bloodstream.
Figure 6. In this TEM image, the basement membrane (BM) delineates the boundaries of the follicle, which contains a thyroglobulin colloid (Co); apical microvilli (Mv) are visible on the apical surface of the thyrocytes, or cuboidal follicular cells (F). The cytoplasm of a C cell (C) can be seen next to a follicular cell, with many electron-dense secretory granules containing calcitonin. Near the lumen of the follicle, vacuoles (V) containing thyroglobulin bound to T3 and T4 fuse with lysosomes (L) to free the hormone for secretion into the capillaries (Cap).
Goiters
In the absence of dietary iodide (for example, iodized salt), reduced levels of thyroid hormone are produced. As a result, there is a corresponding increase in thyroid stimulating hormone (TSH) released by the pituitary gland. With chronic underproduction of thyroid hormones, the continued elevation in TSH stimulates follicular cell hyperplasia and hypertrophy. Increased levels of thyroglobulin also expand the follicular lumen with colloid. The result is an iodine-deficiency goiter. This form of goiter is the most common (accounting for 90%).
Graves Disease
In Graves disease, an autoimmune disorder, auto antibodies activate the TSH receptor and lead to increased thyroid hormone synthesis and secretion. This leads to increased thyroid growth, resulting in the formation of a goiter. This is the most common form of hyperthyroidism, a general condition leading to overproduction of thyroid hormones. This results in symptoms such as weight loss, nervousness, sweating, and heat intolerance. Graves disease is often accompanied by exophthalmos, a protrusion of the eyes due to excess fat and ECM tissue accumulation in the orbit.
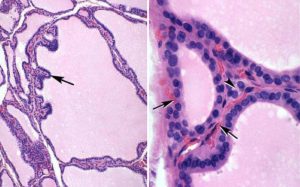
Inappropriate activation of the TSH receptor leads to thyroid hyperplasia and overproduction of thyroid hormone, causing formation of a goiter (top). Because the antibodies also target fibroblasts behind the eye, hyperplasia can also result there, leading to exophthalmos (bottom).
PARATHYROID GLAND
As the name implies, the parathyroid glands are located near to the thyroid, usually along the posterior surface. They are often embedded within the substance of the thyroid (Figure 7). This gland is composed of chief cells and oxyphil cells.
Figure 7. Parathyroid glands are embedded in the
posterior surface of the thyroid lobes.
Chief cells
These are by far the more numerous of the two cell types in the parathyroid and are small cells with dark, centrally located nuclei (Figure 8). These cells produce parathyroid hormone (PTH). This hormone raises blood calcium levels by increasing osteoclast activity and stimulating vitamin D activation in the kidney. Activated vitamin D stimulates calcium resorption in the GI tract. The actions of PTH are opposite to that of calcitonin and the two are both used in maintaining calcium homeostasis (Figure 9), although only PTH is essential for life.
Oxyphil cells
These cells have copious eosinophilic cytoplasm that surrounds dark, centrally located nuclei, and are much larger than chief cells (Figure 8). Often cell-cell borders between
Figure 8. Parathyroid tissue contains two cell types: chief cells (C), which comprise the majority of
parathyroid tissue; and oxyphil cells (O), which may be derivatives of chief cells but have no known function. Parathyroid glands also exhibit a large amount of adipose tissue.
adjacent oxyphil cells are visible. Since oxyphil cells tend to exist in clumps, they are easily distinguished from the chief cells. Oxyphil cells appear at the onset of puberty, but have no known function. Some show low levels of PTH synthesis, which suggests that they may be derivatives of chief cells.
Figure 9. The thyroid and parathyroid release hormones that maintain equilibrium of blood calcium levels by affecting osteoclast activity and calcium deposition in bone.
SUPRARENAL (ADRENAL) GLANDS
The adrenal glands are situated superior to the kidneys and are surrounded by a thick connective tissue capsule (Figure 10). Trabeculae extend inward from this capsule to support the highly cellular glandular tissue. These organs have a distinct cortex and medulla with the cortical region responsible for the production of steroid hormones (derived from cholesterol) and the medullary region responsible for producing catecholamines (derived from the amino acid tyrosine). The cortex is under the control of adrenocorticotropic hormone (ACTH) produced by the anterior pituitary. The medulla is under the control of the sympathetic nervous system and, to a lesser extent, hormones from the adrenal cortex.
Figure 10. The adrenal glands sit on the superior surface of the kidneys, and have a distinct cortex and medulla. The adrenal glands produce a variety of cholesterol- and amino acid-derived hormones that they secrete into the bloodstream.
Adrenal Cortex
The adrenal cortex consists of three layers of cells: an outer zona glomerulosa, a
middle zona fasciculata, and an inner zona reticularis (Figure 11). In addition to these
cells, a rich vascular supply branches throughout the cortex, extending into the medulla.
Cells in the cortex show typical specializations for steroid hormone production, including
lipid droplets, abundant mitochondria and smooth ER.
Figure 11. The adrenal cortex consists of three distinct zones, each of which specializes in production of a particular steroid hormone.
Zona glomerulosa
This outer region is so-named because of the cells’ distribution in small clumps (L. glomus = ball, Figure 12), and it is located just deep to the CT capsule. These cells synthesize and secrete mineralocorticoids, chiefly aldosterone, largely in response to angiotensin II levels. Aldosterone is important for electrolyte and water balance. Its main targets are the distal convoluted tubules of the kidney and striated ducts of salivary glands to regulate sodium resorption.
Zona fasciculata
This middle region is the widest of the three zones, occupying approximately 80% of the cortical volume. It consists of cords of cells that are arranged radially, which are full of lipid precursors; much of the cytoplasm is lost during fixation and consequently appears bubbly (Figure 13). In TEM preparations, the cells have abundant SER and lipid. The main hormones produced are glucocorticoids and sex steroids. Glucocorticoids, principally cortisol, have important roles in carbohydrate, lipid and protein metabolism. Sex steroids (chiefly a variety of androgens) are active in stimulating secondary sex characteristics in males and also serving as the precursor for estrogens.
Figure 12. The zona glomerulosa is the most
superficial layer and secretes primarily
aldosterone, under the influence of angiotensin II,
and affects sodium transport in the kidney.
Zona reticularis
This inner zone is a narrow region adjacent to the medulla and contains cells arranged in small networks or cords (Figure 14). These cells have fewer lipid droplets than the zona fasciculata and thus appear as a distinct layer. These cells secrete mainly androgens.
Figure 13. The zona fasiculata contains abundant lost during H&E preparation. Glucocorticoids are produced in this region under the influence of ACTH.
Figure 14. The deepest layer of the adrenal cortex, the zona reticularis, uses DHEA to produce androgens under the influence of ACTH.
Adrenal Medulla
The inner region of the adrenal gland contains many “chromaffin” cells, so named
because of their affinity for chromium salts. These cells are derived from the neural
crest and are of two types, E-cells and NE-cells. E-cells are much more abundant, and
they store and release the neurotransmitter epinephrine. NE-cells store and release
norepinephrine. These neurotransmitters are also known as adrenaline and
noradrenaline, respectively. Special stains will reveal the two cell populations but even
without them, two cell populations in the medulla are evident (Figure15). When viewed
by electron microscopy, the secretory granules in the NE and E cells are of different
sizes.
Both cell types function in a manner analogous to postganglionic neurons in the
sympathetic nervous system. In the sympathetic nervous system and adrenal medulla,
Figure 15. Chromaffin cells in the adrenal medulla are essentially post-ganglionic cell bodies; preganglionic sympathetic neurons synapse on their membranes. In chromium salt stained preparations, the two types stain differently, revealing the epinephrine-secreting (A) and norepinephrine-secreting (N) subtypes of chromaffin cells. By TEM (right), the two cell types can be distinguished based on the difference in lipid
droplet size: norepinephrine (NE) droplets are larger than those containing epinephrine (E).
preganglionic neurons communicate with postganglionic neurons using the
neurotransmitter acetylcholine. In the case of the sympathetic nervous system, the postganglionic neuron then travels to its target organ and uses the neurotransmitters
epinephrine or norepinephrine to stimulate that target. In the adrenal medulla,
epinephrine or norepinephrine are secreted directly into the blood stream. Thus,
chromaffin cells are effectively modified postganglionic neurons and, when stimulated,
will elicit the same responses as in the sympathetic nervous system (increase in heart
rate, increase in blood pressure, bronchiole dilation, etc.).
The medulla has a dual blood supply, with some coming directly from vessels within the
capsule and some draining into the medulla after passing through the cortex. The latter
is significant in that cortical products, such as glucocorticoids, regulate the medullary
enzyme phenylmethanolamine N-methyltransferase that converts norepinephrine
into epinephrine.
Figure 16. Summary of cell types in the adrenal cortex and the products they secrete.
Feedback/Errata